|
Greetings!
Don't forget to register for our
upcoming Seventh
Conference on Future Energy which gives you
the option of two conferences concurrently:
www.futurenergy.org.
The registration
fee for COFE7 will be going up to $200 on June 1st but the joint,
double conference fee of $400 is the same as the ExtraOrdinary
Technology conference fee and grants access to both conferences.
This month we have several extras
for you. One of our readers, Gary Vesperman, has assembled his
current edition of Gallery of Clean Energy
Inventions (10).pdf which is quite extensive. Also
controversial but worth mentioning is NASA's new development of a
propellantless electromagnetic drive system: Next
Big Future: Inventor Guido Fetta describes EMdrive related
propellentless Cannae drive aka Q drive system.
Apparently what IRI has learned is
that even though the published journal article indicates success in
producing force with the Fetta design, NASA is hoping for at least
ten times the power. Tune in next time for further developments
Also happening in June is the
second International Conference on Nanotechnology, Nanomaterials
and Thin Films for Energy Applications brings together academia,
industry and policy-makers interested in the application of
nanotechnology in the energy sector, and will address new materials
and nanotechnologies for energy harvesting, production, storage,
transfer and other applications.www.nanoenergy.co.uk
Nano Energy Manchester, UK June 1-3.June 2015.
Story #1 is quite
exciting since Elon Musk will be penetrating the domestic market with
his new solar batteries that can power your home and get more
renewables onto the grid, as well as making it cheaper to power your
electric car.
Story #2 offers the
latest MIT breakthrough with bacteria that normally think for
themselves but now are on the payroll for producing human fuel with
sunlight. The goal of highly efficient artificial photosynthesis is a
long-standing one and now genetically engineered E coli have been
coaxed to make certain chemicals with solar energy. This is the first
working example of such a direct interface between bacteria and semiconducting
materials for artificial photosynthesis.
Story #3 is somewhat
related biologically with plants being the center of attention for a
wide range of nanobionic products that have enhanced ability to
capture light for example and exhibit augmented technology. The
possibilities of plant nanobionics are potentially far-reaching. Now
we don't have to limit their applications to just our diet.
Story #4 is a big one
that finally gives the public the long-term plans that NASA has for
conquering the cosmos with fifteen projects that NASA wants to have
funded through its Innovative Advanced Concepts Program. It is quite
an exciting list of science projects, many of which are
energy-related.
Story #5 is a real
breakthrough that is great for California and the rest of the
dehydrating earth with ORNL's effective desalination with graphene.
In what we think is the simplest experiment one could imagine, they
made a screen that has a pore size of 0.5-1 nm in size which is just
big enough for the small H2O to pass through but not the bigger NaCl
molecule and other organic ones too. This should be a school
teacher's dream demo with plastic molecule toys to show how effective
such a design is. The high pressure reverse osmosis nightmare seems
to have been solved with graphene's high flow (high flux)
possibilities now but the salt rejection needs to be improved
slightly to be commercially useful.
THOMAS VALONE
Integrity Research Institute
|
|
OUR LATEST CONFERENCE
COFE7
JULY 30-AUG 1, 2015
ALBUQUERQUE , NM
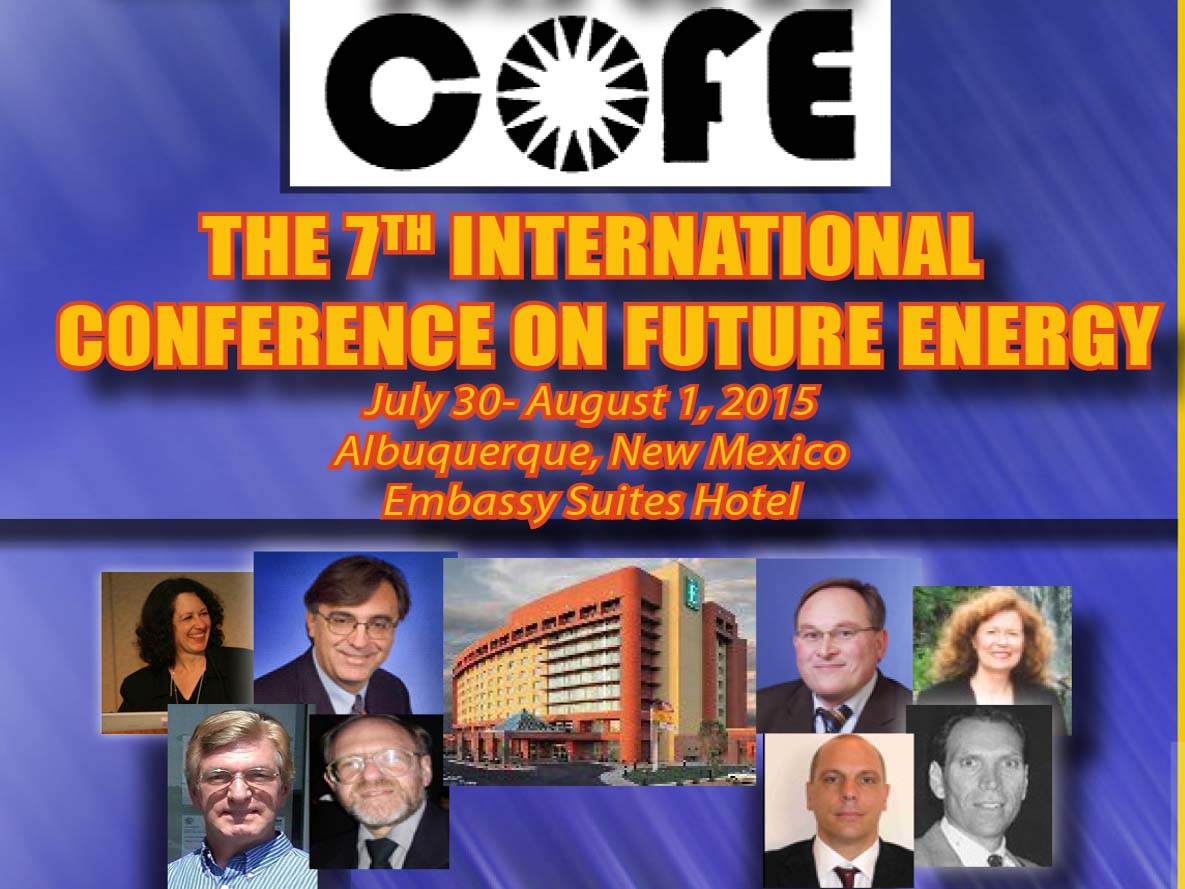
Osteoporosis? Our Newest Item
OsteoPad
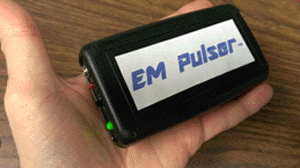
EM
Pulser
Our best selling PEMF device
PREMIER
Jr.
Our best selling Tesla coil device
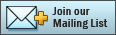
|
|
|
1) Tesla's Elon Musk Shows off Solar Batteries
to Create a Revolution
|
By Chris Mooney, Washington Post, May 2,
2015
http://www.washingtonpost.com/news/energy-environment/wp/2015/04/30/why-teslas-announcement-could-be-such-a-big-deal/?tid=pm_pop_b
Late Thursday,
the glitzy electric car company Tesla Motors, run by billionaire Elon
Musk, ceased to be just a car company.
As widely expected, Tesla announced that it is offering a
home battery product, which people can use to store energy from their
solar panels or to backstop their homes against blackouts, and also
larger scale versions that could perform similar roles for companies
or even parts of the grid.
For homeowners,
the Tesla Powerwall will have a power capacity of either 10
kilowatt hours or 7 kilowatt hours, at a cost of either $ 3,500 or $
3,000. The company says these are the costs for suppliers and don't
include the cost of installation and a power inverter, so customers
could pay considerably more than that.
The battery,
says Tesla, "increases the capacity for a household's solar
consumption, while also offering backup functionality during grid
outages."
At the same
time, the company said it will producing larger batteries for
businesses and utility companies - listing projects with Texas-based
Oncor and Southern California Edison.
[Powering your home with batteries is going to get
cheaper and cheaper]
The anticipation
leading up to this announcement has been intense - words like "zeitgeist" are being used - which
itself is one reason why the moment for "energy
storage," as energy wonks put it to describe
batteries and other technologies that save energy for later use, may
finally be arriving. Prices for batteries have already been dropping, but if Tesla adds a "coolness factor" to the equation,
people might even be willing to stretch their finances to buy
one.
The truth,
though, is Tesla isn't the only company in the battery game, and
whatever happens with Tesla, this market is expected to grow. A study by GTM Research and
the Energy Storage Association earlier this year found that while
storage remains relatively niche - the market was sized at just $128
million in 2014 - it also grew 40 percent last year, and three
times as many installations are expected this year.
[Why your next home might be battery powered]
By 2019, GTM
Research forecasts, the overall market will have reached a size of $
1.5 billion.
"The trend
is more and more players being interested in the storage
market," says GTM Research's Ravi
Manghani. Tesla, he says, has two unique advantages - it is
building a massive battery-making "gigafactory" which should
drive down prices, and it is partnered with solar
installer Solar City (Musk is Solar City's chairman), which
"gives Tesla access to a bigger pool of customers, both
residential and commercial, who are looking to deploy storage with or
without solar."
The major upshot
of more and cheaper batteries and much more widespread energy storage
could, in the long term, be a true energy revolution - as well as a
much greener planet. Here are just a few ways that storage can
dramatically change - and green - the way we get power:

1. Helping to
integrate more renewables onto the grid.
Almost everybody
focusing the Tesla story has homed in on home batteries - but in
truth, the biggest impact of storage could occur at the level of the
electricity grid as a whole. Indeed, GTM Research's survey of the
storage market found that 90 percent of deployments are currently at
the utility scale, rather than in homes and businesses.
That's probably
just the beginning: A late 2014 study by the Brattle
Group, prepared for mega-Texas utility Oncor, found that energy
storage "appears to be on the verge of becoming quite
economically attractive" and that the benefits of deploying
storage across Texas would "significantly exceed costs"
thanks to improved energy grid reliability. Oncor has proposed spending as much as $
5.2 billion on storage investments in the state. California, too, hasdirected state utilities to start
developing storage capacity - for specifically environmental reasons.
For more power
storage doesn't just hold out the promise of a more reliable grid -
it means one that can rely less on fossil fuels and more on
renewable energy sources like wind and, especially, solar, which
vary based on the time of day or the weather. Or as a 2013
Department of Energy report put it, "storage can
'smooth' the delivery of power generated from wind and solar
technologies, in effect, increasing the value of renewable
power."
"Storage is
a game changer," said Tom Kimbis, vice president of executive
affairs at the Solar Energy Industries Association, in a statement.
That's for many reasons, according to Kimbis, but one of them is that
"grid-tied storage helps system operators manage shifting peak
loads, renewable integration, and grid operations." (In
fairness, the wind industry questions how much storage will
be needed to add more wind onto the grid.)
Consider how
this might work using the example of California, a state that
currently ramps up natural gas plants when power demand increases at
peak times, explains Gavin Purchas, head of the Environmental Defense
Fund's California clean energy program.
In California,
"renewable energy creates a load of energy in the day, then it
drops off in the evening, and that leaves you with a big gap that you
need to fill," says Purchas. "If you had a plenitude of
storage devices, way down the road, then you essentially would be
able to charge up those storage devices during the day, and then
dispatch them during the night, when the sun goes down. Essentially
it allows you to defer when the solar power is used."
This will be
appealing to power companies, notes Purchas, because "gas is
very quick to respond, but it's not anywhere near as quick as
battery, which can be done in seconds, as opposed to minutes with
gas." The consequences of adding large amounts of storage to the
grid, then, could be not only a lot fewer greenhouse gas emissions,
but also better performance.
2. Greening
suburban homes and, maybe, their electric cars, too.
Shifting away
from the grid to the home, batteries or other forms of storage have
an equally profound potential, especially when paired with rooftop
solar panels.
Currently,
rooftop solar users are able to draw power during the day and, under net metering arrangements, return some
of it to the grid and thus lower their bills. This has led to a great
boom in individual solar installations, but there's the same problem
here as there is with the grid as a whole: Solar tapers off with the
sun, but you still need a lot of power throughout the evening and
overnight.
But storing
excess solar power with batteries, and then switching them on once
the solar panels stop drawing from the sun, makes a dramatic
difference. Homes could shift even further away from reliance on the
grid, while also using much more green power.
Moreover, they'd
also be using it at a time of day when its environmental impact is
greater. "If you think about solar, when it's producing in the
middle of the day, the environmental footprint is relatively
modest," explains Dartmouth College business professor Erin
Mansur. That's because at this
time of day,
Mansur explains, solar is more likely to be displacing electricity
generated from less carbon intensive natural gas. "But if you
can shift some of that to the evening ... if you can save some to the
middle of the night, it's more likely to be displacing coal,"
says Mansur.
Some day,
perhaps, some of the sun-sourced and power could even be widely used
to recharge electric vehicles like Teslas - which would solve another
problem. According to a much discussed 2012 paper by Mansur and two
colleagues, electric vehicles can have a surprisingly high energy
footprint despite their lack of tailpipe emissions because
they are often charged over night, a time when the power
provided to the grid (said to be "on the margin") often
comes from coal.
But if electric
vehicles could be charged overnight using stored power from the sun,
that problem also goes away.
All of
which contributes to a larger vision outlined recently
by a team of researchers at the University of
California at Los Angeles's Institute of the Environment and Sustainability
in which suburban homeowners, who can install rooftop solar combined
with batteries and drive electric vehicles, start to dramatically
reduce their carbon footprints - which have long tended to be bigger
in suburbia, due in part to the need for long commutes - and also
their home energy bills.
[How solar power and electric cars could make
suburban living awesome again]
Granted, it's
still a vision right now, rather than a reality for the overwhelming
number of suburbanites - but energy storage is a key part of that
vision.
3. Helping
adjust to smart energy pricing
And there's
another factor to add into the equation, which shows how energy
storage could further help homeowners save money.
For a long time,
economists have said that we need "smart" or
"dynamic" electricity pricing - that people should be
charged more for power at times of high energy demand, such as in the
afternoon and early evening, when the actual electricity itself costs
more on wholesale markets. This would lead to lower prices overall,
but higher prices during peak periods. And slowly, such smart pricing
schemes are being introduced to the grid (largely on a voluntary
basis).
But if you
combine "smart" pricing with solar and energy storage, then
homeowners have another potential benefit, explains Ravi Manghani of
GTM Research. They could store excess power from their solar panels
during the day, and then actually use it in the evening when prices
for electricity go up - and avoid the higher cost. "There's an
economic case to store the excess solar generation and use it during
evening hours," explains Manghani by email. (For more
explanation, see here.)
Notably, if
there are future reductions in how much money solar panel owners can
make selling excess power back to the grid - and that's one
thing the current pushback against net metering wants to achieve -
then energy storage comes in and gives panel owners a new way for
using that power.
"Storage
increases the options," explains Sean Gallagher, vice president
of state affairs at the Solar Energy Industries Association.
"It's an enabling technology for solar. It allows customers to
meet more scenarios economically."
So in sum -
cheaper, more easily available energy storage helps at the scale of
the power grid, and also at the level of our homes, to further
advantage cleaner, renewable energy. So if the economics of storage
are finally starting to line up - and its business side to ramp up -
that can only be good news for the planet.
Correction: A
previous version of this article stated (based on a report from GTM
Research) that the energy storage market grew 400 percent in 2014 and
was sized at $ 139 million - and expected to be worth $1.4 billion in
2019. Those figures have been updated, and the correct figures
are 40 percent, $ 128 million, and $ 1.5 billion.
|
2) Bacteria
Produces Fuel with Sunlight
|
By Mike Orcutt, MIT Technology Review, May 2015
http://www.technologyreview.com/news/537051/how-nanomaterials-can-help-make-fuel-from-sunlight/
Researchers say combining bacteria with nanoscale
semiconductors opens a new path toward efficient artificial
photosynthesis.
Researchers at
the University of California, Berkeley, say that by combining nanoscale
materials with bacteria, they have opened the door to a new way of
designing systems that could efficiently turn carbon dioxide, water,
and sunlight into useful organic compounds-similar to what plants do
through photosynthesis. Down the road, they say, the system could
become a commercially viable way to produce high-value chemicals like
drug precursors used by the pharmaceutical industry, or to store
renewable energy in the form of liquid fuels.
The goal of
highly efficient artificial photosynthesis is a long-standing one,
and there are many approaches to the problem, all of which face
scientific hurdles (See "Sun + Water = Fuel" and "A Greener 'Artificial Leaf'").
One general approach is to rely on microörganisms called electrotrophs,
which can be coaxed, through the application of electricity, to make
certain chemical building blocks.
The new system
is the first one in which semiconductors, which are capable of both
capturing solar energy and transmitting electricity to the microbes,
have been directly combined with bacteria, says Peidong Yang, a professor of chemistry
and materials science at the University of California, Berkeley, and
an inventor of the system. Previous similar systems have relied on
bulky solar panels to provide renewable electricity (see "Making Diesel from CO2 and Sunlight").
In this case, semiconducting nanowires capture energy from sunlight
and pass electrons to electrotrophic bacteria, which are nestled
within the wires. The electrotrophs use the electrons to turn carbon
dioxide and water into useful chemical building blocks. Those are
then passed to genetically engineered E. coli, which in turn
make a wide range of products.
This is the
first working example of such a direct interface between bacteria and
semiconducting materials for artificial photosynthesis, says Yang. He
and his colleagues demonstrated that the system
could make butanol, a polymer used in biodegradable plastics, and
three pharmaceutical precursors. It could in principle be used to
make many other products, including chemicals that are valuable in
relatively small volumes-unlike fuel, which must be produced at a
very large scale to be economical.
The new system
is about as efficient as natural photosynthesis at using the energy
in sunlight, says Yang. That's not enough for the process to be
commercially viable, but he says new semiconductor materials his
group is currently working with should make the process more
competitive. "Efficiency is something we can improve in the near
future," he says.
An important
potential advantage of this particular design, besides the
light-capturing nanowire array, is that it can be used in the
presence of oxygen, says Eric Toone, a professor of
biochemistry and chemistry at Duke University and former director of
ARPA-E's electrofuels program, which focuses on developing
technologies that use electrotrophic organisms to make fuel (the
program funded Yang's group while Toone was at the helm). The
well-studied bacteria Yang's group used cannot naturally tolerate
oxygen, which has made the organism difficult to use at a large
scale, says Toone. In the new design, says Yang, the nanowires
"protect" the bacteria from oxygen.
Still,
microbe-based systems face significant challenges because the
bacteria must be kept alive, and even at best they don't live very
long. And compared with chemical catalysts, bacteria are slow
"engines," says Nate Lewis, a professor of chemistry
at Caltech.
Indeed, Yang
says his team's ultimate goal is a synthetic system that is more
stable than the bacteria-based system. But at the moment, he says,
there are no better catalysts than bacteria for converting carbon
dioxide into useful compounds. He and his colleagues are now looking
closely at the way in which the semiconducting materials transfer
electrons to the microbes. Investigating this semiconductor-bacteria
interface could yield useful insights toward the design of a
synthetic catalyst that could replace the bugs.
|
3) Turning
Plants into Technology
|
By Michael Strano, Juan Pablo Giraldo, Physics
World, May 2015,
http://live.iop-pp01.agh.sleek.net/physicsworld/reader/#!edition/editions_nanotechnology-2015/article/page-6886
Nanomaterials
are being used to give plants novel and augmented functions, with
potential applications ranging from self-healing materials to living
electronics
Nanobionic plant: An Arabidopsis plant with carbon nanotubes inside
its leaves could have an enhanced capability to capture light and
thus act as a photonic biochemical detector. (Juan Pablo
Giraldo)
Imagine that you were to visit a distant planet
and find its surface blanketed with sophisticated machines. These
machines sense and respond to their environment, diagnose and repair
themselves, and create their own fuel from their surroundings. As a
technology-savvy earthling, you would be incredulous to learn that
the only value seen in such machines was to grind them up, process
and eat them - yet this is precisely what we do every day. Plants on
Earth possess all of these diverse functions and more, but only now
are we beginning to consider the potential of plants for new
technologies.
Augmented technology
The nascent field of "plant
nanobionics" seeks to harness known properties of plants to
augment or reinvent human technology. By treating living plants as
technological platforms we can learn how to integrate nanoparticles
with plant-based materials to impart novel functions to devices.
Sensors in the form of plants could sample their environment through
transpiration and report the result via radio-frequency signals, for
example, or we can imagine self-repairing, plant-based photonic
devices that serve as communications networks. Plants even have their
own power source - photosynthesis, which has the added benefit of
consuming carbon dioxide - and are made of cheap materials that are
naturally recyclable.
The possibilities of plant nanobionics are potentially
far-reaching. A world in which materials repair themselves using
sunlight or where buildings in cities act as active carbon sinks
would be transformative. Nanoelectronic devices parasitically wired
and integrated into a plant's internal machinery could draw power,
store energy and communicate sensory information relating to water
stress, chemical exposure, nutrient stress or ambient illumination.
How realistic is this vision? While there are definite limitations to
plant nanobionics, this is a new field with much unexplored territory
and scope for surprise.
Consider photosynthesis from an engineering
perspective. A plant chloroplast (the photosynthetic engine of the
organism) can produce sugars at an average rate of 40 µg per
square centimetre per hour. That is equivalent to 10% of the energy
stored in a watch battery each day - not enough to power your
smartphone or tablet, but sufficient to drive an active
radio-frequency identification circuit to export information, an
electrochemical sensor to generate information, or a luminescent
beacon for signalling.
The stems of plants, which transport sap from
the roots to the leaves via a bundle of conduits each being
10-100 µm across, are another natural engineering marvel that
could be exploited for devices. The electrolyte-containing conduits
have an electrical conductivity of 0.5 millisiemens per centimetre,
which is more than 30 times higher than that of silicon at room
temperature, therefore providing channels for parallel communications
from the ground to the tips of leaves.
Furthermore, pressure drops induced in the
xylem from evaporating water inside leaves can reach values of more
than -3 MPa. This is similar in magnitude to the pressure drops that
power the entire field of microfluidic devices, which today are
typically provided by bulky external pumps.
These are just a few examples that reveal the
potential of plants as engineering materials, but there is much more
infrastructure within the plant that could be tapped for
applications. Plant nanobionics is distinct from the now
well-established field of biomimetics, in which engineers learn from
natural systems to create new synthetic materials, because it seeks
to incorporate living plants into the final device. In plant systems,
the use of nanotechnology for this purpose has no precedent.
Merging disciplines
Working at the intersection of plant physiology
and nanotechnology, we became interested in plant nanobionics in 2010
thanks to a research project in which we studied plant self-repair
mechanisms during photosynthesis. Our goal was to mimic such repair in
synthetic devices, and we successfully built self-assembled
photo-electrochemical devices by combining carbon nanotubes into a
plant's photosystem. However, the project motivated us to investigate
whether we could exploit existing functions of the plant's natural
machinery - such as self-repair and the conversion of solar energy
into fuels - to create high performance, self-repairing solar cells.
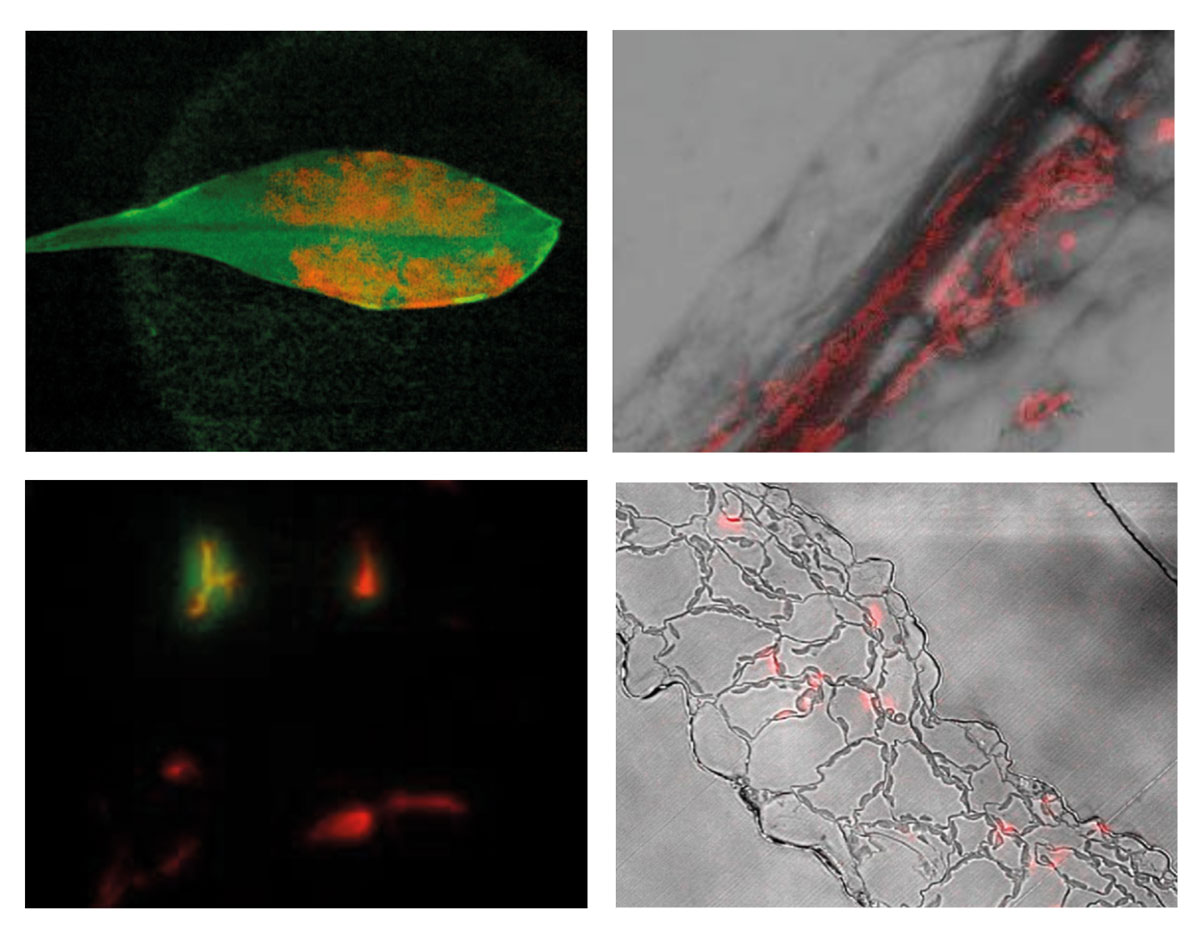
Merging materials
In vivo fluorescent imaging of a plant
that has been integrated with single-walled carbon nanotubes (red),
showing their presence in (clockwise, from top left): the leaf, near
leaf veins, parenchyma cells and chloroplasts. (Nature Materials)
Last year we reported a series of new
techniques that allow nanoparticles to be delivered and localized
within living plants and plant organelles, and we were able to
demonstrate several novel functions that could emerge as a result (Nature Materials 13 400).
Using extracted spinach chloroplasts and leaves
from Arabidopsis plants, we found that single-walled carbon
nanotubes (SWCNTs) augment both the light reactions of photosynthesis
and biochemical detection functions in these species. Furthermore, we
discovered that, under certain conditions, SWCNTs can be made to
assemble within the chloroplast's photosynthetic machinery, which has
proven to be a powerful enabling technique.
Surprisingly, SWCNTs that are thousands of times longer
than the thickness of a lipid bilayer penetrate the outer lipid
envelopes of chloroplasts and are left trapped on the inside. Wrapped
in highly charged molecules, the SWCNTs assemble within the
chloroplast photosynthetic machinery via a mechanism we call lipid
exchange envelope penetration (LEEP): the SWCNTs become coated with
lipids that form the chloroplast envelopes, pulling nanotubes in as
the membrane repairs itself and thereby trapping them inside. LEEP
could potentially be used to make many new types of hybrid
photosynthetic materials in plants, including those that absorb light
at wavelengths across the electromagnetic spectrum or those that have
chemo-protective capabilities against photo-damage. We demonstrated
that SWCNTs can enhance the rates of photo-induced electron transport
both in chloroplasts extracted from the plant-cell host and in leaves
of living plants by up to 30% relative to controls.
The delivery of SWCNTs to living plants was performed by
infiltration through the stomata, which are the pores that control
gas exchange between leaves and the atmosphere. We also exploited
this mechanism to deliver nano-sized particles of cerium oxide (known
as "nanoceria") inside chloroplasts, significantly reducing
the levels of reactive oxygen species to values 28% lower than in
control chloroplasts. Nanoceria particles act catalytically as potent
scavengers of these damaging molecules, a bit like supercharged
vitamin C, thus protecting the chloroplast protein complexes and
significantly extending the lifetime of the plant.
We also showed that plants assembled with
carbon nanotubes can act as chemical sensors that communicate by
fluorescent signalling. These nanobionic plants report changes in the
concentration of nitric oxide (a plant-signalling molecule and
environmental pollutant) by modulating the near-infrared emission of
nanoparticle sensors embedded within the leaf lamina. The modified
plants are able to respond within seconds of exposure and are capable
of detection sensitivities below one part per million in aqueous
media. We envision such nanobionic plants replacing more expensive
inorganic sensors based on electronics and plastics for the detection
of explosives or environmental pollutants, for instance.
The discovery that SWCNTs can assemble within a
plant's photosynthetic machinery raises the possibility of
biocompatible electrodes patterned within and on the surfaces of
leaves and stems. These could be interfaced with plant tissues to
produce electrical circuits for computation, electrochemical
detection of molecules inside the plant, or external communication.
This merging of synthetic and natural infrastructures is one of the
central visions of plant nanobionics. A laccase-glucose oxidase
electrode pair, for example, creates a biofuel cell that could syphon
off stored glucose to electrically power the circuits. This could
allow the circuit to monitor the plant's photosynthetic output
directly by quantifying the sugars that are the main products of
photosynthesis.
Monitoring other plant signals is similarly
intriguing. Abscisic acid, for instance, is a hormone produced by
roots in response to dry soil conditions that controls plant
transpiration by closing the stomatal aperture. We can therefore
imagine nanoelectronic circuits that respond to plant chemical
signals and control the water content of their environment, for
example by generating a radio-frequency signal that activates an
irrigation device in response to water stress.
We can even consider incorporating plant
systems directly into our own building materials to provide added
functionality. Since chloroplasts can perform the basic function of
converting sunlight and carbon dioxide into sugars even when they are
removed from a living plant cell, materials containing transplanted
chloroplasts could potentially capture unwanted carbon dioxide from
the atmosphere. For this to be possible, however, we first need to
prevent the natural degradation of the "naked" chloroplasts
caused by reactive oxygen species and other mechanisms when they are
removed from the plant cell. At the Massachussetts Institute of
Technology we have recently been working on the concept of a
"hyperstable chloroplast" as an engineering material,
perhaps based on chemo-protective nanoparticles such as nanoceria.
Calling all physicists
Such visions might at first seem a bridge too
far. Plant nanobionics requires interdisciplinary research teams and
strong collaboration between plant scientists and nanotechnology
researchers to make it reality, but there is a wealth of scientific
knowledge and technological potential to be gained on the way towards
this goal. Enhancing crop yields and the productivity of algae
biofuel, or creating novel hybrid photovoltaic and optical
communication materials, are already widely studied technological
goals. But some applications, such as authentic plant cyborg tissue,
require completely new avenues of exploration.
There are many different scientific and
engineering challenges for plant nanobionics in the decade ahead. We
have shown that nanoparticles introduced to a plant can be trafficked
to the chloroplasts in leaves via vascular infusion, but what about
directing other nanoparticles to other plant organs or tissues to
boost or introduce additional functions? Does a given nanoparticle
with particular properties and coatings affect its transport within
the plant? Such questions are still poorly understood, but will help
to develop plant biocompatible circuits and optical communication
materials.
To bridge the world of electronics and plants,
we also need to understand the physical limitations imposed by the
plant. We have to determine, for example, how electromagnetic
interactions within and between nanomaterials affect the way that
visible, infrared or radio-frequency waves interact with living
plants. As with every new technology, safety studies should also be
thoroughly conducted before taking nanobionic plants outside the
laboratory. Nanotoxicity studies demonstrate that the behaviour of
nanomaterials in living tissue depends a lot on the surface chemistry,
aspect ratio, nanoparticle size and other properties. While studies
in this area will help engineers to design additional biocompatible
materials for the plant interface, many nanobionic applications -
such as those designed to replace or enhance silicon, plastic and
metal devices for communications, photonics or self-powered systems -
do not involve ingestible nanoparticles.
There are seemingly endless opportunities and
challenges in using nanotechnology to enhance and exploit the diverse
functions of plants. One certainty, however, is that plant
nanobionics requires the engagement of multidisciplinary teams of
plant biologists, chemists, engineers and physicists alike.
Michael Strano is a chemical engineer and Juan
Pablo Giraldo is a plant physiologist at the Massachusetts Institute
of Technology, US, e-mailstrano@mit.edu
back to table of contents
|
4) 15 Projects that NASA Wants to
Change from Science Fiction to Science Fact
|
By Mika Mikinnon, Space.com May 5, 2015
http://space.io9.com/15-projects-nasa-wants-to-change-from-science-fiction-t-1703362134

The projects
funded by NASA Innovative Advanced
Concepts program sound more like a list of science
fiction dreams than plausible research, yet that's exactly what they
are. These 15 projects just received $100,000 to explore how feasible
they can be.
Several
of the projects involve innovative uses for small, compact, low-cost
satellites called CubeSats that can carry limited payloads, or rely
on alternative energy sources to reduce dependence on nuclear power for
space exploration.
1. Wind-Powered Drone Pairs For More Efficient
Atmospheric Research Platforms
The Virtual Flight Demonstration
of Stratospheric Dual-Aircraft Platform will link a
pair of glider drones with a cable as they soar around the
stratosphere, providing a long-term atmospheric platform. Led by
William Engblom at Embry-Riddle Aeronautical University, the project
will deploy aircraft powered by wind shear that get an extra boost
from solar films and possibly even a wind turbine. The aircraft will
be paired at different altitudes (up to a kilometer apart) so they're
in significantly different wind regimes. The upper glider, SAIL,
provides lift and aerodynamic thrust, while the lower aircraft,
BOARD, provides upwind force. This should give a substantial power
boost over traditional solar
aircraft, allowing for multi-year stationkeeping and
long-term platforms for earth observation or communication.
2. New Liquid Capture For More Efficient Air
Scrubbing
Keeping air
clean is a major problem in contained environments like space
stations and submarines. The Thirsty Walls - A new paradigm for air
revitalization in life support project is being developed under the
direction of John Graf at NASA Johnson Space Center to swap out forced-air
systems with liquid capture instead. Forced air is annoying because
it's complicated, requires a lot of moving parts, restricts airflow,
and in microgravity, also require heavy, inefficient removal beds.
Early-generation liquid capture systems required gas permeable
membranes, which were both slow and tended to get poisoned over time.
This new technology uses capillary fluid mechanics to directly expose
cabin air as passive "curtains" that don't require high
pressure or high flow velocity. It's also a step up from submarine
systems, replacing Monoethanolamine with ionic liquid as the CO2
capture for better power efficiency.
3.
Pulsar-Based Navigation System For Deep Space Missions
The A Tall Ship
and a Star to Steer Her By is being developed by Massachusetts
Institute of Technology's Michael Hecht. Along with joining the list
of absurd astronomy acronyms with Differential Deployable Autonomous
Radio Navigation, or DARN, the project wants to use radio
observations of quasars, pulsars, and masers as navigational beacons
for deep space missions. If it works, this could be the
interplanetary version of GPS for navigation. For this early phase,
the project is just putting together a catalogue of sources and
design concept for how to run a technology demonstration mission.
4. Rocket
Fuels That Can Be Mined In Space
The In-Space Manufacture of Storable Propellants wants to solve a
basic problem: how to provide propellent for space missions without
wasting yet more propellent while getting that propellent into space.
Instead of shipping propellent from Earth into orbit, Principle
Investigator is John Lewis of Deep Space Industries is trying to find
ways to manufacture propellent in space. A major challenge with
rocket fuel is to make it storable so it only explodes upon request:
we use a hydrazine fuel with a N2O4 oxidizer. The problem with mining
volatiles from Near-Earth Asteroids is a lack nitrogen, so the
proposal will need to develop an alternate suitable storable
oxidizing agent.
5. Tiny CubeSats To Poke At The Composition Of
Asteroids And Comets
Joseph Wang is leading the charge
on the CubeSat with Nanostructured Sensing Instrumentation for
Planetary Exploration, a project mixing the excitement of cheap, tiny
CubeSats with our growing expertise at landing on comets and
asteroids. The key part of the project are cheap, lightweight,
compact, disposable sensors being developed at the University of
Southern California and the University of Utah that can detect 74
trace elements to the nearest part per billion (ppb). If the TiO2
nanotube sensing platform can be successfully integrated into
CubeSats, they open up the possibility of being able to ground-truth
our remote sensing of the composition of the small rocky and icy
bodies of our solar system.
6. Mini-Seismic Surveys To Investigate The Interior
Structure Of Asteroids
The Seismic Exploration of Small
Bodies project tickles my geophysical heart by
bringing seismic surveys to tiny lumps of rock and ice in deep space.
Under Jeffrey Plescia at Johns Hopkins University, the project will
combine micro-seismometers developed at Arizona State University with
CubeSats to create impactors to investigate the interiors of asteroids
and comets. The concept is very simple: drop at least one
micro-seismometer on the target's surface, then smack it with a
projectile as a seismic energy source to produce a known signal. The
seismic data could be interpreted using the same inversion techniques
as seismic surveys here on Earth, providing data on the seismic
velocity (thus interior structure) of asteroids and comets.
7. Directed Energy Propulsion for Interstellar
Exploration
Wants to up our game with interstellar exploration by
advancing the next generation of deep space probes. Phil Lubin's
research group at the University of California at Santa Barbara is
looking at pairing directed energy propulsion with wafer-scale
spacecraft to create tiny probes propelled by phased arrays of lasers.
The miniature satellites will be designed to supplement the
long-range remote sensing currently done by orbital telescopes. While
initially interplanetary explorers, the wafer satellites could
theoretically be boosted to relativistic speeds and be our first
interstellar probes.
8. Rocket-Powered Hopper To Explore Neptune's Moon
Triton
The DEEP IN
Directed Energy Propulsion for Interstellar Exploration wants to up
our game with interstellar exploration by advancing the next
generation of deep space probes. Phil Lubin's research group at the
University of California at Santa Barbara is looking at pairing
directed energy propulsion with wafer-scale spacecraft to create tiny
probes propelled by phased arrays of lasers. The miniature satellites
will be designed to supplement the long-range remote sensing
currently done by orbital telescopes. While initially interplanetary
explorers, the wafer satellites could theoretically be boosted to
relativistic speeds and be our first interstellar probes.
9. Submarine Squid
To Explore The Oceans Of Europa
The development of the Soft-Robotic Rover with
Electrodynamic Power Scavenging is being led by Mason
Peck of Cornell University. The soft, squid-inspired robot would be
the first submarine rover to explore another planet. The planned
power systems are all about taking advantage of the local environment:
the tentacles will harvest power from changing magnetic fields. In
turn, the tentacles will power electrolysis to separate water into
hydrogen and oxygen gas. The gas will be used to inflate the squid,
changing its shape to propel it through fluids. Europa is
the most famous watery moon that could be explored by this squid, but
it could also work on other moons of Jupiter and Saturn that have liquid lakes or oceans.
10. Robot Swarm
To Explore Lunar Shadows For Volatile Elements
The CRICKET: Cryogenic Reservoir Inventory by
Cost-Effective Kinetically Enhanced Technology being developed by
Jeffrey Plesia at Johns Hopkins University is all about bouncing
around the darkest slivers of the moon. A small herd of robots will
explore perpetually shadowed regions on the lunar poles for water and
other volatile elements. The swarms consist of three roles: a swarm
of crickets to hop, crawl, and roll whike exploring the shadows; a
carrier hive to collect data, navigate, provide power, and disperse
the crickets on the surface; and an orbiting queen to deliver the
robots and provide communication. The robots are all extensions of
existing technology, although these particular variants will carry
spectrographs, lamps, heating elements, and whiskers to characterize
the volatiles.
11.
Supercooling Materials To Provide Radiation Sheilding And Energy
Storage
NASA Kennedy Space Center's Robert Youngquist is the principle
investigator for Cryogenic Selective Surfaces, a project to develop
surfaces for extreme passive cooling. By creating materials with with
wavelength-dependent emissivity and absorption properties, the
research team is hoping they can create new cryogenic storage and
large-scale superconducting systems that can be used in deep space
for galactic cosmic radiation shielding or energy storage. The
prototype materials have been tested on Earth to cool to -50°C below
ambient temperatures, but could theoretically work much better in a
vacuum.
12.
Sunlight-Drills To Capture And Mine Asteroids For Water
The APIS (Asteroid Provided In-Situ
Supplies): 100MT Of Water from a Single Falcon 9 is the idea of Joel
Sercel of ICS Associates Inc to fix the problem of how to find usable
water in space in an affordable, accessible manner. The team hopes
that they can wrap asteroids in bags, then use optical mining to
concentrate sunlight to drill into them. The project is designed to
be lightweight and compact enough that all the equipment can be
loaded unto a single rocket launch (Falcon 9 or equivalent),
harnessing the technology of the Asteroid Redirect Mission to capture
a target and trap outgassing water released during optical mining.
13.
WindBots To Explore The Cloudy Skies Of Gas Giants
The WindBots: persistent in-situ
science explorers for gas giants is exactly what it
says on the label: a project to create autonomous robots that can
investigate the atmospheres of Jupiter, Saturn, Uranus, or Neptune.
Under the guidance of Jet Propulsion Laboratory's Adrian Stoica, the
project is hoping to design robots that can directly harvest energy
locally, allowing them to persistently explore their assigned gas
giant. That same technology could theoretically be applied to other
planetary robotic explorers, reducing their reliance on expensive
nuclear energy.
14. Deformable Mirrors Shaped By Magnetic Fields
Melville Ulmer at Northwestern
University is partnering with researchers at the University of
Illinois to investigate the feasibility of creating shapable
telescope mirrors with magnetic fields.Aperture: A Precise
Extremely large Reflective Telescope Using Re-configurable Elements is
a concept that combines a flying magnetic write head with magnetic
smart material coating the back of a mirror, creating a deformable
reflecting membrane. Earlier iterations of the concept ran into
problems with distorting the mirror outside of correctable
error-bounds, and creating a mirror that can keep its shape for long
periods of time.
15. New Type Of Lens To Reduce The Cost Of Large
Telescopes
One of the most expensive things
about building telescopes is developing beautiful, flawless lenses to
focus light. Nelson Tabirian is leading the Thin-Film Broadband Large
Area Imaging System project at BEAM Engineering for Advanced
Measurements Co. to apply their waveplate lens technology to creating
a new type of light-weight, economical thin film lens. The waveplate
lenses and mirrors could theoretically be used to build telescopes
with a far larger aperture than currently feasible under current
technology and economic considerations, leading to a new generation
of ultra-enormous telescopes. The technology uses techniques
developed for laser communication to correct chromatic aberrations,
permitting submicroradian angular radiation.
back to table of contents
|
5) Using
Graphene For Desalination
|
By Matthew Chalmers Physics World, May 2015
http://live.iop-pp01.agh.sleek.net/physicsworld/reader/#!edition/editions_nanotechnology-2015/article/page-6858
A team at the
Oak Ridge National Laboratory (ORNL) in the US has demonstrated
desalination technology using free-standing, porous graphene
membranes. Seawater desalination is usually performed via
reverse-osmosis polymer-based filters, which require significant
pressure to push water from one side to the other.
By offering a
more porous and thinner membrane, explains study co-leader Shannon
Mark Mahurin of the ORNL, graphene could increase the flux and
achieve the same purification rate with a smaller membrane area.
"That all serves to reduce the amount of energy that it takes to
drive the process," he says.
Water molecules
are too big to pass through graphene's fine mesh, so the team made
holes using a silicon-nitride stencil and an oxygen plasma that
knocked carbon atoms out of the 2D hexagonal lattice.
The resulting
membrane allowed water - but not salt ions - to penetrate, with the
optimum pore size for effective desalination found to be
0.5-1 nm (Nature
Nanotechnology 10.1038/nnano.2015.37). "It's a huge
advance," says Mahurin. "The flux through the current
graphene membranes was at least an order of magnitude higher than
[that through] state-of-the-art reverse-osmosis polymeric
membranes."
Menachem
Elimelech of Yale University in the US, who was not involved in the
study, says that the challenge for graphene-based membranes is to
scale them up to larger areas, and warns that seawater desalination
demands a salt-rejection level of greater than 99.9%.
"This work
represents progress in the development of graphene-based desalination
membranes, but it shows a much lower salt rejection," Elimelech
told Physics World. "It is now well known that high-flux
membranes will not reduce the energy of seawater desalination because
the transport of water through the membrane is governed to a large
degree by the osmotic pressure, and further graphene membranes will
need to be tested under hydraulic pressures, as in real
operation."
back to table of contents
|
About Integrity Research Institute
Future Energy eNews is
provided as a public service from Integrity Research Institute,
a Non-Profit dedicated to educating the public on eco-friendly
emerging energy technologies.
FREE copy
of the 30 minute DVD "Progress in Future Energy" is
available by sending an email with
"Free DVD" in subject and mailing address in
body.
Your generous support is
welcome by making a tax deductible donation on
our secure website
|
|
|
|
|
|